Cheap, reliable energy has made the modern world possible. Where once humans and animals burnt calories to provide the raw power to perform work, machines now burn fuels or consume electricity in their place. The typical human can continuously and comfortably produce approximately 75 Watts (W) of useful power each hour. A 18650 type lithium-ion battery can store about 9.62W of power, while a gallon of gasoline contains about 33,000W. With the move to unreliable wind and solar generation systems, inexpensive and reliable methods must be developed and deployed to cover shortfalls when environmental conditions do not favor power generation by wind or solar. Shortfalls may be covered by things such as natural gas peaker plants or energy storage systems. Because gas-powered plants are often opposed by proponents of wind/solar, the remaining option is to consider energy storage.
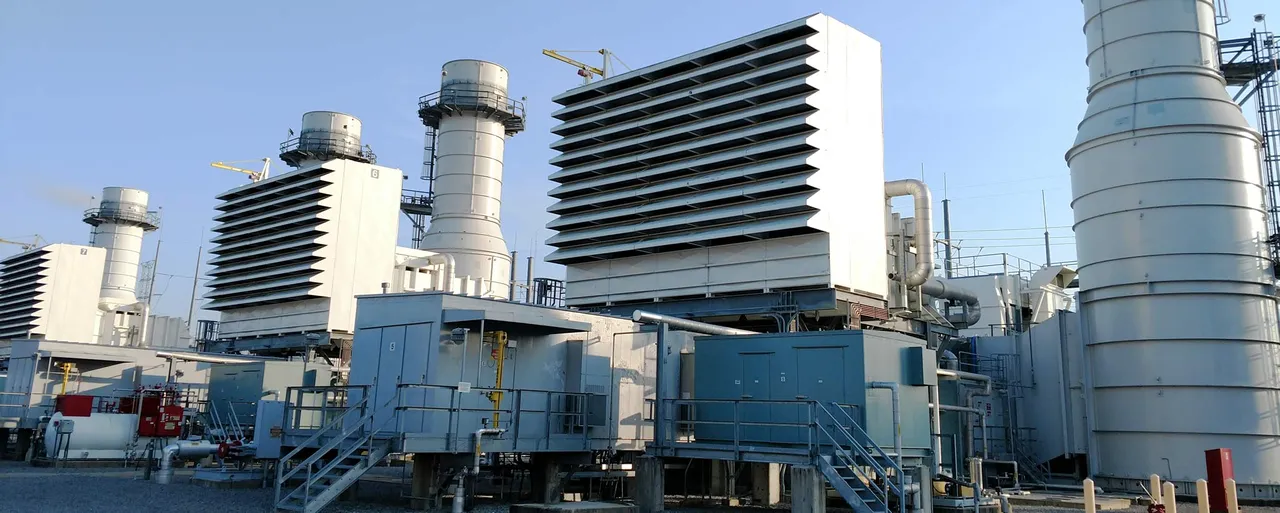
TVA's Marshall combustion turbine "peaking" plant
Several excellent energy storage mechanisms exist in nature, for example, animals store excess energy as fat and plants often store it as sugar. However, there is currently no method in widespread use to store excess electrical energy. When demand for electrical power falls, power plants supplying the grid must shunt off the excess energy until the plant's output can be matched to the demand. In the case of a coal or oil-fired plant, this excess energy is often released as steam. In the case of wind, one or more wind turbines are brought a stop. In solar power installations, segments of the installation are disconnected from the grid. Three viable options are currently in use to store large amounts of electricity.
The oldest option is pumped-storage, which uses excess electricity to pump water into reservoirs, where it can later be released through a hydroelectric generation system. The second is electrochemical, where excess power is used to charge large banks of batteries. Finally, a much newer technology uses excess electricity to create methanol which can later be burned in an internal combustion engine to create power. When discussing energy storage, it is important to understand that all storage systems consist of three components. The first of these is a mechanism to convert electricity to some form that can be stored. The second is the storage system itself. The third component is a method to convert or recover energy from the storage medium.
As previously stated, pumped-storage operates by using excess electricity to pump water from a lower elevation to a reservoir at a higher elevation. When demand for electricity exceeds generation, water held in the reservoir is released through hydroelectric turbines. The National Hydropower Association (NHA), a non-profit whose membership consists of North American companies in the hydroelectric power business, estimates there is over 20 gigawatts (GW) of pumped-storage available in the U.S., with an additional 31GW proposed to cover for new wind and solar installations. Pumped-storage has the advantage of being able to use the same turbines to pump water into a reservoir that generate electricity by releasing water from the reservoir. The biggest disadvantages to pumped-storage are that it can typically only be used in areas with the right topography to create a reservoir, and the reservoir consumes land which may be used for other purposes.
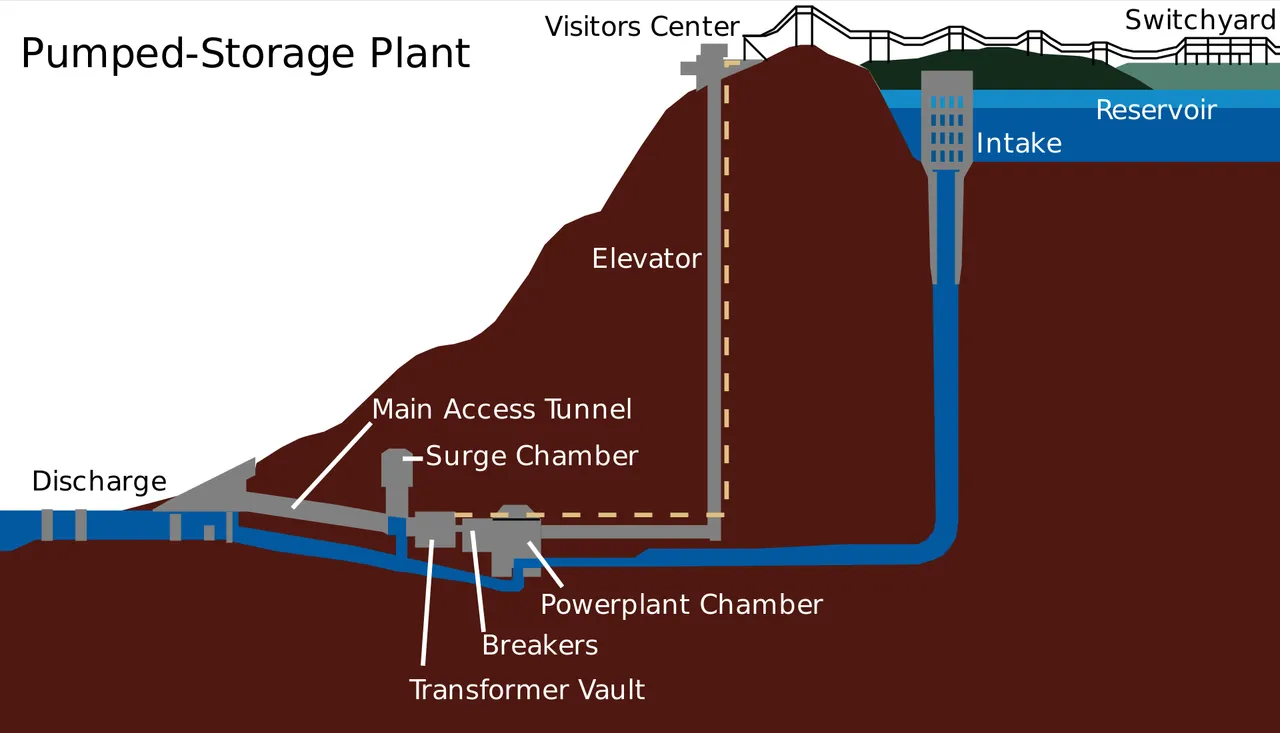
Raccoon Mountain pumped-storage diagram
The electrochemical cell, which stores energy in chemical bonds between metals and oxidizers, has been in use for over a century. More commonly known as batteries, there are several rechargeable designs in popular use today, including lead-acid, nickel-metal hydride, and lithium-ion. Lead-acid batteries are the least expensive, requiring only lead and sulfuric acid, and are most often found as sources of backup power in telephone exchanges, power plants, military infrastructure, and hospitals. Lithium-ion batteries offer a much greater energy density than lead-acid chemistries and are usually the primary sources of power for mobile devices including laptops, cellphones, and electric vehicles.
To use batteries as large-scale energy storage devices, anywhere from several dozen to thousands of batteries are connected in an arrangement that permits maintenance operations while minimally impacting the operation of the storage system. Energy to charge the batteries is usually provided by electricity from a power distribution network, and energy stored in the batteries can be sent back out to the distribution network when needed). This arrangement is known as grid storage. More often, however, this stored energy is used by the owner of the storage system as a source of power when the distribution network goes down. There are two modes of operation when this happens, the most common being battery-backup until on-site diesel-powered generators can supply power, and the other being battery-backup that supplies all power a site needs. There are also several grid-storage projects being developed, the largest being Ruien Energy Storage’s (RES) site in Ruien, Belgium. RES is a Belgian partnership formed by two companies, Belgium’s Yuso and Japan’s Nippon Koei. In a 2018 press release, RES confirmed plans to construct, on the site of a decommissioned 800MW coal-fired power plant, a 25MW battery-based storage system with room for expansion.
Batteries are great methods to store electrical energy. They have no moving parts resulting in relatively rugged devices. They are also safe, as long as the storage and operational limitations for a particular battery chemistry are observed. However, when batteries are stored or operated outside of their specifications, they may emit poisonous gasses or start intense fires. Lithium presents a special problem because it can be burned in water, breaking the chemical bonds between water’s oxygen and hydrogen molecules, combining with the oxygen in an energetic oxidation reaction, and releasing hydrogen. Hydrogen, in turn, is highly flammable in the presence of oxygen. Conventional firefighting methods using water would only add fuel to a fire where lithium is present in amounts required for battery-based energy storage systems. Brownell Limited, a UK-based desiccant manufacturer, hosted a short video demonstrating the reaction when a lithium battery is placed into water.
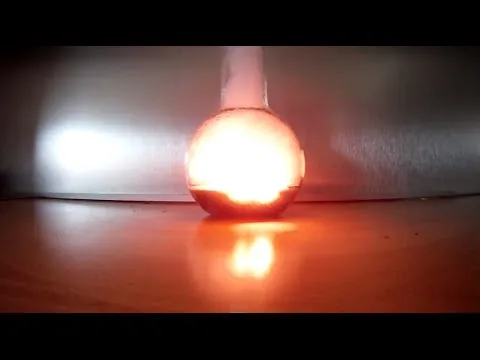
In the video, a lithium battery is dropped into a glass vessel containing water and ignites in a high-energy exothermic reaction. Lithium-ion batteries also present dangers to firefighters and/or others during fires or when the batteries have been damaged. A 2016 journal article written by Jesse Roman of the National Fire Protection Association (NFPA) covered two examples of these sorts of problems. In the first example, a battery ignited three weeks after being damaged in a crash test conducted by the National Highway Transportation Safety Administration, and in the second example a battery reignited 22 hours after it was extinguished in a test conducted by the Fire Protection Research Foundation.
The final energy storage technology to be examined in this paper is power-to-liquid, where electricity is used to create hydrocarbons like methanol, gasoline, kerosene, and diesel. In these processes, CO2 is combined with hydrogen obtained from electrolysis to form hydrocarbons. Teknisk Ukeblad (TU. English: Technical Weekly Magazine) is a Norwegian engineering magazine created by a partnership consisting of three Norwegian engineering firms. A press release written by TU journalist Jannicke Nilsen, and endorsed by Nordic Blue Crude, stated that approximately 100 million liters of diesel could be made using 200MW of electricity at Nordic Blue Crude’s future power-to-liquid plant.
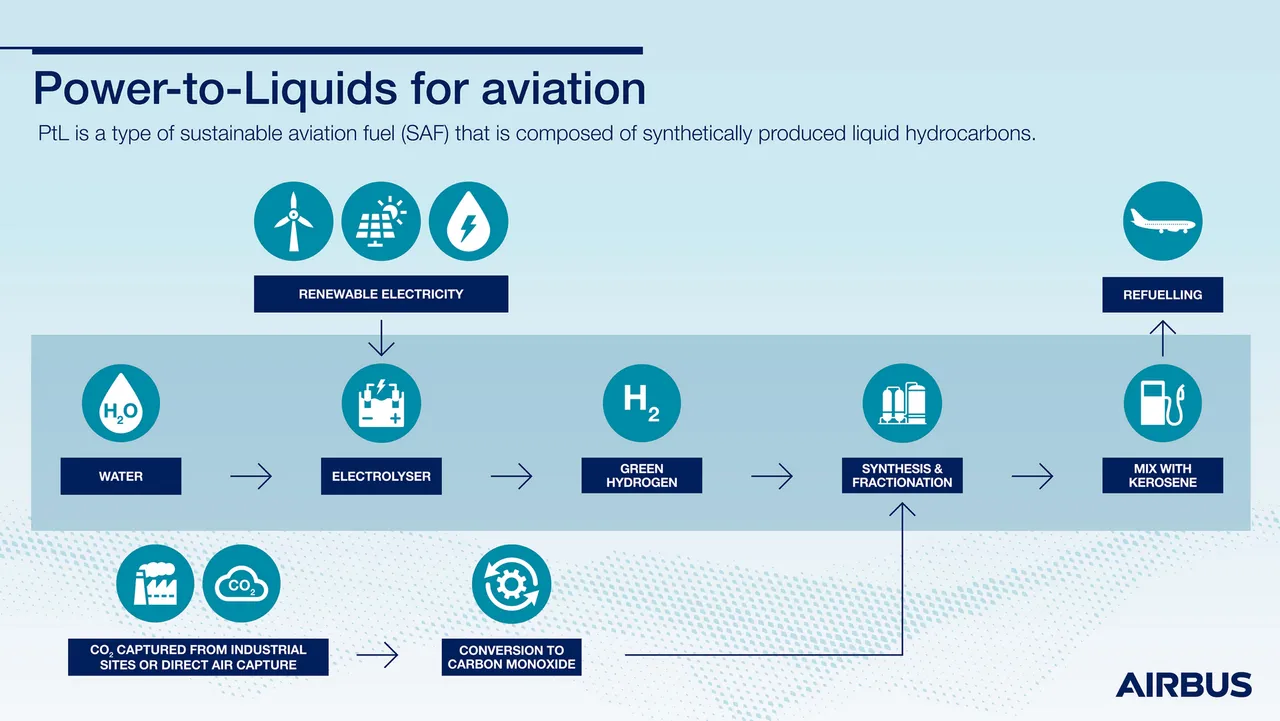
The advantages of power-to-liquid over electrochemical storage are numerous. The storage medium itself requires no hard-to-recycle minerals like lithium. A large and well-developed infrastructure already exists to safely transport and store liquids like gasoline and kerosene. Today’s firefighters are already well-trained and equipped to deal with fires involving fuels like gasoline, in which the fire can often be extinguished by overwhelming it with water. Water is readily available from fire hydrants, lakes, rivers, and oceans and is also inexpensive.
One key advantage of power-to-liquid is the high energy density and energy-to-mass ratio (specific energy) relative to electrochemical storage systems. A 2012 American Physical Society (APS) article written by Fred Schlachter, a physicist working at the Lawrence Berkeley National Laboratory, briefly compared the energy density/specific energy of gasoline and batteries. According to Schlachter, gasoline can store about 100 times more energy than a comparable lithium-ion battery. Even when inefficiencies with automobile internal combustion engines are accounted for, about five times more energy can be extracted from gasoline than from batteries.
While gasolines, diesel, and other hydrocarbons are vastly better storage mediums than batteries, the process of rectification is much more efficient than converting electricity to hydrocarbons. Rectification-regulation is the process of converting the alternating current (AC) of power grids to direct current (DC) needed to charge batteries The efficiency of rectifiers was analyzed in an article written by Dave Divins, a senior field engineer for International Rectifier Corporation. Divins concluded a properly designed rectifier-regulator converts about 94.5% of the AC power put into it to DC power. Additional energy losses are encountered in charging batteries, but they are negligible. To send energy stored in batteries to an electrical grid, the DC must be converted back in to AC using inverters. Divins also analyzed power inverter designs and found the conversion efficiency of DC to AC can range from 85% to 95% depending on various factors. Both conversion processes result in a conversion efficiency of about 85.9% on average.
Even though power-to-liquid suffers from low conversion efficiencies, it can have a place in a society that is worried about CO2 emissions. Aviation is one example of such a place because of the requirements to keep aircraft lightweight and maximize available space. Kerosene coupled with turbine engines have been used in aviation for decades to provide the power needed to make aircraft fly. According to the Air Transport Action Group, an international organization whose membership is composed of companies in the aviation sector, all the world’s aircraft emitted 859 million tonnes (about 947 million tons) of CO2 in 2017. It might not be unreasonable to see most aviation fuel produced by power-to-liquid plants that remove CO2 from the atmosphere, thus negating any CO2 emissions from aircraft.
Ships are another example where space and/or weight requirements tend to determine how a ship will be propelled through the water. With about 4.5 million gallons of fuel oil, an average container ship carries with it about 190,526,941,800W of power. 4.5 million gallons of fuel oil weighs approximately 17,257 tons. For comparison, powering a ship using 18650-type lithium-ion batteries would require 19,846,556,438 batteries with a total mass of 1,050,100 tons. This is more than the weight of any fully loaded container ship today. As with aviation, power-to-liquid could negate CO2 emissions from ships.
Power-to-liquid can also address violations of property Rights. One such example is the push by some governments to ban vehicles with internal combustion engines. The City of London is one example where traditionally-powered vehicles have been banned in a test-run to evaluate the effects of such bans. If CO2 emissions are the concern, power-to-liquid can negate these emissions and preserve the property rights of those who owned traditionally-powered vehicles before such bans go into place.